
May 11, 2022
By MARCELLO
ROSSI
The Race to Produce Green Steel
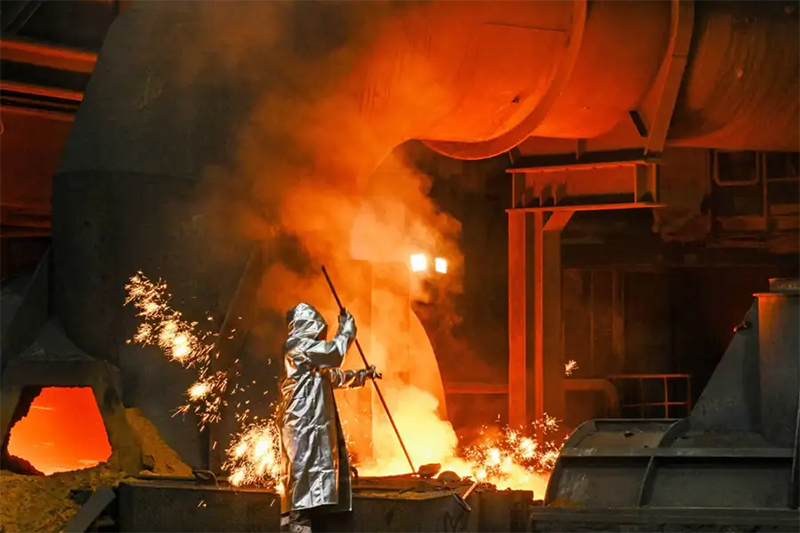
Top: Tapping Boston Metal’s semi-industrial molten oxide electrolysis
cell. Visual: Boston Metal
IN THE CITY of Woburn, Massachusetts, a suburb
just north of Boston, a cadre of engineers and scientists in white
coats inspected an orderly stack of brick-sized, gunmetal-gray steel
ingots on a desk inside a neon-illuminated lab space.
What they were looking at was a batch of steel created using an
innovative manufacturing method, one that Boston Metal, a company that
spun out a decade ago from MIT, hopes will dramatically reshape the
way the alloy has been made for centuries. By using electricity to
separate iron from its ore, the firm claims it can make steel without
releasing carbon dioxide, offering a path to cleaning up one of the
world’s worst industries for greenhouse gas emissions.
An essential input for engineering and construction, steel is one of
the most popular industrial materials in the world, with more than 2
billion tons produced annually. This abundance, however, comes at a
steep price for the environment. Steelmaking accounts for 7 to 11
percent of global greenhouse-gas emissions, making it one of the
largest industrial sources of atmospheric pollution. And because
production could rise by a third by 2050, this environmental burden
could grow.
That poses a significant challenge for tackling
the climate crisis. The United Nations says significantly cutting
industrial carbon emissions is essential to keeping global warming
under the 1.5 degrees Celsius mark set under the 2015 Paris climate
agreement. To do so, emissions from steel and other heavy industries
will have to fall by 93 percent by 2050, according to estimates by the
International Energy Agency.
Facing escalating pressure from governments and investors to reduce
emissions, a number of steelmakers — including both major producers
and startups — are experimenting with low-carbon technologies that use
hydrogen or electricity instead of traditional carbon-intensive
manufacturing. Some of these efforts are nearing commercial reality.
“What we are talking about is a capital-intensive, risk-averse
industry where disruption is extremely rare,” said Chris Bataille, an
energy economist at IDDRI, a Paris-based research think tank.
Therefore, he added, “it’s exciting” that there’s so much going on all
at once.
Still, experts agree that transforming a global industry that turned
over $2.5 trillion in 2017 and employs more than 6 million people will
take enormous effort. Beyond the practical obstacles to scaling up
novel processes in time to reach global climate goals, there are
concerns about China, where over half the world’s steel is made and
whose plans to decarbonize the steel sector remain vague.
“It’s certainly not an easy fix to decarbonize an industry like this,”
said Bataille. “But there’s no choice. The future of the sector — and
that of our climate — depends on just that.”
MODERN STEELMAKING INVOLVES several production
stages. Most commonly, iron ore is crushed and turned into sinter (a
rough solid) or pellets. Separately, coal is baked and converted into
coke. The ore and coke are then mixed with limestone and fed into a
large blast furnace where a flow of extremely hot air is introduced
from the bottom. Under high temperatures, the coke burns and the
mixture produces liquid iron, known as pig iron or blast-furnace iron.
The molten material then goes into an oxygen furnace, where it’s
blasted with pure oxygen through a water-cooled lance, which forces
off carbon to leave crude steel as a final product.
This method, first patented by English engineer Henry Bessemer in the
1850s, produces carbon-dioxide emissions in different ways. First, the
chemical reactions in the blast furnace result in emissions, as carbon
trapped in coke and limestone binds with oxygen in the air to create
carbon dioxide as a byproduct. In addition, fossil fuels are typically
burned to heat the blast furnace and to power sintering and
pelletizing plants, as well as coke ovens, emitting carbon dioxide in
the process.
As much as 70 percent of the world’s steel is produced this way,
generating nearly two tons of carbon dioxide for each ton of steel
produced. The remaining 30 percent is almost all made through electric
arc furnaces, which use an electrical current to melt steel — largely
recycled scrap — and have far lower CO2 emissions than blast furnaces.
“It’s certainly not an easy fix to decarbonize an industry like this,”
said Bataille. “But there’s no choice. The future of the sector — and
that of our climate — depends on just that.”
But because of the limited scrap supply, not all future demand can be
met this way, said Jeffrey Rissman, an industry program director and
head of modeling at the San Francisco-based energy and climate policy
firm Energy Innovation. With the right policies in place, recycling
could supply up to 45 percent of global demand in 2050, he said. “The
rest will be satisfied by forging primary ore-based steel, which is
where most emissions come from.”
So “if the steel industry is serious” about its climate commitments,
he added, “it will have to fundamentally reshape the way the material
is made — and do so fairly quickly.”
ONE ALTERNATIVE TECHNOLOGY being tested replaces coke with hydrogen.
In Sweden, Hybrit — a joint venture between the steelmaker SSAB, the
energy supplier Vattenfall, and LKAB, an iron ore producer — is
piloting a process that aims to repurpose an existing system called
direct reduced iron. The process uses coke from fossil fuels to
extract oxygen from iron ore pellets, leaving a porous iron pellet
called sponge iron.
The Hybrit method instead extracts the oxygen using fossil-free
hydrogen gas. The gas is created through electrolysis, a technique
that uses an electric current — in this case, from a fossil-free
energy source — to separate water into hydrogen and oxygen. (Most pure
hydrogen today is made with methane, which produces CO2 when burned.)
The resulting sponge iron then goes into an electric arc furnace,
where it’s eventually refined into steel. The process releases only
water vapor as a byproduct.
“This technology has been known for a while, but it’s only been done
in the lab so far,” said Mikael Nordlander, head of industry
decarbonization at Vattenfall. “What we are doing here is to see if it
can work at [the] industrial level.”
Last August, Hybrit reached its first milepost: SSAB, which produces
and sells the end product, delivered its first batch of fossil-free
steel to the automaker Volvo, which used it in vehicle prototypes. It
is also planning a plant for commercial-scale production, which it
aims to complete by 2026.
Another Swedish venture, H2 Green Steel, is
developing a similar commercial-scale hydrogen steel plant with the
help of $105 million raised from private investors and companies
including Mercedes-Benz, Scania, and IMAS Foundation, an organization
linked to Ikea. The company plans to begin production by 2024 and
produce 5 million tons of zero-emissions steel annually by the end of
the decade. Other companies testing hydrogen-powered steelmaking
include ArcelorMittal, Thyssenkrupp, and Salzgitter AG in Germany;
Posco in South Korea; and Voestalpine in Austria.
Electricity can also be used to reduce iron ore. Boston Metal, for
example, has developed a process called molten oxide electrolysis, in
which a current moves through a cell containing iron ore. As
electricity travels between both ends of the cell and heats up the
ore, oxygen bubbles up (and can be collected), while iron ore is
reduced into liquid iron that pools at the bottom of the cell and is
periodically tapped. The purified iron is then mixed with carbon and
other ingredients.
“What we do is basically swapping carbon for electricity as a reducing
agent,” explained Adam Rauwerdink, the company’s senior vice president
of business development. “This allows us to make very high-quality
steel using way less energy and in fewer steps than conventional
steelmaking.” As long as power comes from fossil-free sources, he
added, the process generates no carbon emissions.
He said the company, which currently runs three pilot lines at its
Woburn facility, is working to bring its laboratory concept to the
market, using $50 million raised last year from an investor group
including Breakthrough Energy Ventures, backed by Bill Gates, and the
German carmaker BMW. A commercial-scale demonstration plant is
expected to be up and running by 2025.
“I feel all these solutions have their place, depending on location,
resource availability, and targeted product,” said Sridhar Seetharaman,
a professor of materials science and engineering at Arizona State
University. “However I do not think for now any one alone will give
you a silver bullet to meet the demand.”
“Hydrogen has a bit of a head start being based on an established
system and it’s also ahead in commercialization,” said Bataille, the
IDDRI energy economist. “But achieving a net-zero steel industry will
take more carbon-free pathways, so I think there will be enough room
in the market for all of them in the end.”
ALTHOUGH GREENER STEELMAKING processes appear to be gaining momentum,
there remain a number of serious challenges to confront. Chief among
them is the massive expansion in renewable energy infrastructure that
an industry-wide shift to these new methods would entail, said Thomas
Koch Blank, senior principal at the Colorado-based nonprofit Rocky
Mountain Institute. He estimates that the world would need up to three
times the currently installed solar and wind energy sources to
electrify the existing primary steel production.
Another barrier is cost. Switching to electricity or hydrogen would
require vast amounts of capital spending to erect new plants and
retrofit old ones. In the case of the clean hydrogen method, the price
tag for steel will increase largely because steel producers are
located close to low-cost coking coal rather than low-cost hydrogen,
pointed out Koch Blank. “These upfront costs will likely drive up the
price of both steel and the end products, at least in the beginning.”
According to Rissman, the analyst in San Francisco, legislation on
both the supply and the demand side could help offset those higher
costs and encourage more investment in greener technologies.
Governments, he said, could incentivize the use of low-carbon steel
for building and infrastructure by requiring state-funded projects to
use low-carbon versions of designated construction materials. They
could also enforce policies that make it more expensive to buy from
countries where rules on emissions are less stringent. That will help
domestic producers “stay competitive” as the market for clean steel
“grows and new production processes achieve economies of scale,” said
Rissman.
“It’s a fantastic challenge that we’re up against,” Rauwerdink said.
But, he added, “We are showing that solutions exist — and work.”
Perhaps the biggest roadblock is China, where about 90 percent of
steel production is achieved using blast furnaces. In September 2020,
President Xi Jinping announced that the country aims to become carbon
neutral by 2060. In a bid to reduce pollution from domestic steel
mills, which account for roughly 15 percent of the nation’s overall
carbon emissions, Beijing has also pledged to achieve peak steel
emissions by 2030. Even so, 18 new blast-furnace projects were
announced in China just in the first six months of 2021, according to
the Helsinki-based research group Center for Research on Energy and
Clean Air.
Steel is one of the most important and challenging industries to
decarbonize, said Rissman, so global coordination on it would help
greatly.
Back in Boston, Rauwerdink, surveying Boston Metal’s factory lines,
agreed. “It’s a fantastic challenge that we’re up against,” he said.
But, he added, “We are showing that solutions exist — and work.”
Green Play Ammonia™, Yielder® NFuel Energy.
Spokane, Washington. 99212
www.exactrix.com
509 995 1879 cell, Pacific.
Nathan1@greenplayammonia.com
exactrix@exactrix.com
|